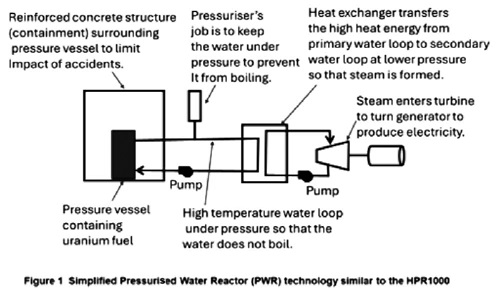
Reflections on nuclear power agreement with China
We, the authors of the book, The Quest for Nuclear Power in Ghana (published by DigiBooks, 2022), have followed with keen interest, the April 24, 2024 news coverage of the Co-operation and Framework Agreement between the Ghana Ministry of Energy and the China National Nuclear Corporation (CNNC) for the construction of an HPR 1000 Technology Nuclear Power Project.
Advertisement
News accounts say the agreement was approved by cabinet, and was signed on the margins of the 26th World Energy Congress in Rotterdam, Netherlands, by Energy Minister, Dr Matthew Opoku Prempeh, and the CNNC President, Mr Yu Jianfeng.
The merits of nuclear power are overwhelming; what most people worry about are the safety issues, thus, in this article we provide a basic understanding of the technology used in HPR 1000 and how the safety issues have been addressed.
However, it is obvious that Ghana is currently not in a sound economic position to proceed with the purchase of a nuclear power plant, so, the most likely possibility for “purchasing” a plant of this size is via the vehicle of BOOT (Build, Own, Operate and Transfer), such as, was done with the AMERI power plant, which is now owned by Ghana, and part of which has been reinstalled in Kumasi.
Nuclear
In general, a nuclear power plant is a device that generates tremendous amount of heat to boil water to steam. The high-pressure steam is used to turn a turbine connected to a generator to generate electricity.
The source of heat (that is, the fuel) is typically uranium, whose atoms have the unique ability to spontaneously break apart, knocking other atoms and breaking them apart also. This process of spontaneous breaking apart of atoms is referred to as “fission.”
A lot of heat is generated during this fissioning process. While this is the basic process for generating heat in a nuclear power reactor, the two well-proven techniques for carrying the heat from the reactor core to generate steam are Pressurised Water Reactors (PWRs) and Boiling Water Reactors (BWRs). [Both PWRs and BWRs use water for the generation of the steam so they are collectively referred to as Light Water Reactors (LWRs)].
Since the HPR 1000 uses PWR technology, we will focus on this technology. Figure 1 shows a simplified diagram of a PWR. The reader is referred to the annotations on the figure for a better understanding of how the PWR generates electricity.
HPR 1000 plant
The HPR 1000 is what is referred to as a Generation III PWR (Gen III PWR). At this point it is helpful to explain what this means. The earliest prototype reactors were developed in the United States in the 1940s and 1950s and are referred to as First Generation (Gen I) nuclear power plants.
Commercial plants followed from the 1960s through the 1980s and are referred to second generation (Gen II) plants. The majority of nuclear power plants still in operation today are Gen II plants.
However, since the early 1990s LWRs have undergone evolutionary improvements. These include inherently-safe operation or improved safety features, greater protection against severe accidents, etc.
As an example, one measure used in assessing a nuclear reactor’s robustness against accidents is the core damage frequency (CDF). This is the likelihood of an accident that would cause damage to the reactor core (see blue area in Figure 1) during the reactor’s operating life. For Gen III and III+ plants, the CDF has been estimated at between 10 and 100 times (depending on the design) lower than that for Gen II plants.
This means that Gen III and Gen III+ plants are 10 to 100 times less likely to have an accident that will cause damage to the reactor core during the operating life of the reactor. Now let us examine how nuclear power plants in general, and the HPR 1000 in particular, is designed to first try to prevent accidents from happening, and in the event that they do happen, how the plant is designed to mitigate them.
First, control the reactor to prevent it from over-generation of neutrons (power) during normal operation; second, remove heat from the core if lack of reactivity control or other factors causes the core to overheat; third, limit the release of radioactivity in case of an accident, such as if there is a large break in the primary piping and heat cannot be removed from the core fast enough; fourth, ensure that heat can be removed from the spent fuel at all times.
As for Gen II plants, the HPR 1000 has design features that addresses all the above. However, the HPR 1000 goes beyond that by using Gen III design methods to achieve a much greater level of reliability and robustness and safety, such as the reduction in core damage frequency mentioned above.
Some of the methods used in the design include the following: the use of redundancy; use of diversity; and the use of independence. The above measures are all part of a defence-in-depth (DiD) approach to the design of a nuclear power plant which, based on a study of the available information on the HPR 1000, have been employed in the design of this nuclear plant.
(DiD design philosophy is defined as the creation of multiple independent and redundant layers of protection and response to failures and accidents.) We turn our attention back to the BOOT type of contract alluded to earlier, and the matter of radioactive waste disposal.
Opinion
We emphasise that we do not know the actual type of the contract under which this project will be implemented. We can only state our opinion that the BOOT type of contract is the only one that makes sense, given that there is absolutely no money to buy it ourselves under any other type of contract.
However, there are downsides to a BOOT contract too. Because the other party is providing all the resources, there is the possibility of raising utility costs to recoup investments, potential refusal to hire Ghanaians in management positions, any lack of conscious technology transfer, etc.
The BOOT contract also requires greater scrutiny and regulatory oversight to safeguard Ghana's interests. A long-term BOOT contract may also be prone to all kinds of oversight requirements "falling through the cracks."
Advertisement
This may be especially true when government changes hands, with attendant change of oversight leadership. Given the importance of such an investment, we hope that there will be long-term oversight, regardless of which government is in power.
The issue of what to do with the spent fuel is also one of the big issues in operating a power reactor anywhere, but especially in a developing country such as Ghana. Why is there spent fuel in the first place?
Spent nuclear fuel is the fuel (uranium rods) that has been operating in the reactor for a long time until it is no longer useful for sustaining the nuclear fission chain reaction in the reactor.
Spent fuel is thermally hot as well as highly radioactive. They are typically stored in spent fuel pools at the reactor site for long periods of time. However, a place for long-term storage must eventually be found. In a developing country such as Ghana with non-existent facilities for any long-term storage, it is the opinion of the authors that the eventual transportation of the spent fuel to the vendor country (China in this case) should be part of the agreement.
Advertisement
The writers are Dr Kofi Korsah (Oak Ridge National Laboratory (retired) and CEO of KERSL Automation); Eng. Laala Anum Kofi (IAEA retired)
Prof.J.H. Amuasi (Director-General of the Ghana Atomic Energy Commission (retired).